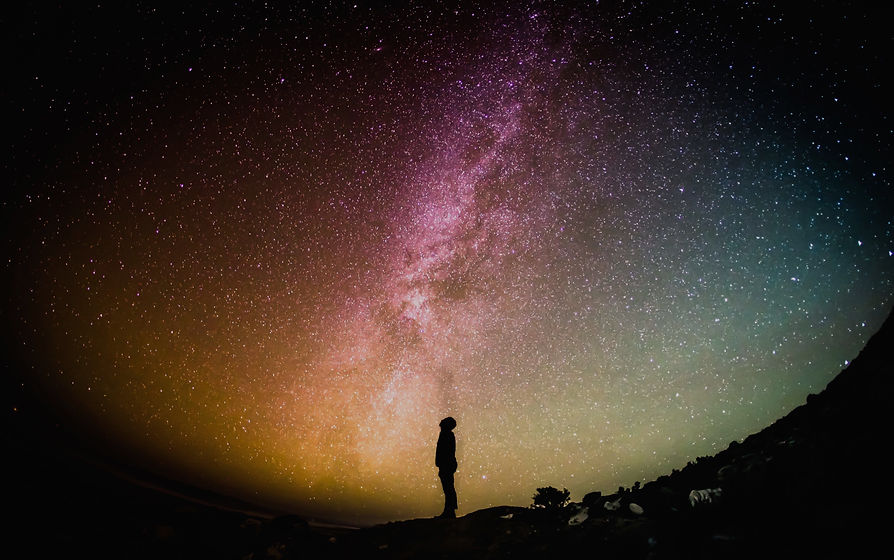
“The joy of discovery is certainly the liveliest that the mind of man can ever feel”
- Claude Bernard -
MY RESEARCH
Plasma is considered the fourth state of matter. Neon lights, lightning and electric sparks are some notable examples of matter in the plasma state that we encounter in our everyday lives. However, here on Earth plasmas are relatively rare and they mostly need to be created in the laboratory. It is instead in outer space where plasmas become predominant and much more common than solids, liquids and neutral gases. In fact, it is often said that plasmas make about the 99% of the visible matter in the Universe. Planetary magnetospheres, stars like our sun, stellar coronae and winds, the interplanetary space and the interstellar medium are only a few examples of plasma environments that can be found in nature. Being highly electrically conductive, plasmas interact strongly with magnetic and electric fields giving rise to complex, inherently nonlinear and multi-scale dynamics. Through their interactions with electromagnetic fields, plasmas provide the mechanisms that power solar activity (and possibly of any star) and most of the energetic phenomena occurring throughout the Universe – from high-energy particle acceleration to geomagnetic storms to disruptive instabilities in confinement fusion devices.
My research adopts analytical and numerical tools together with in-situ data analysis to understand the physics of the solar corona and of the solar wind. I am also interested in fundamental problems of nonlinear plasma dynamics, magnetic field reconnection, and in the thermodynamics of non-collisional plasmas.
From top to bottom: solar flare; northern lights; plasma globe
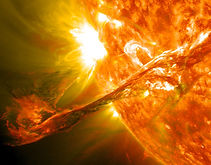
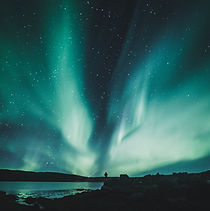
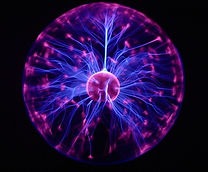
PROJECTS
Turbulence in the inner heliosphere with Parker Solar Probe​
​
The solar wind is permeated by large amplitude fluctuations of plasma velocity and magnetic field known as Alfvénic fluctuations. These Alfvénic fluctuations show features proper of a turbulent medium, such as a developed turbulent energy spectrum, but at the same time they display the correlation that characterizes waves propagating away from the sun. Understanding how this turbulent and yet coherent state is achieved and what is its role in coronal heating and solar wind acceleration remains a longstanding problem of Heliophysics. Even more interestingly, sometimes the magnetic field is so strongly perturbed to the point that it folds back on itself, forming local magnetic field polarity reversals known as "switchbacks". The mystery deepens with Parker Solar Probe which, during its first perihelion at a distance of 35.7 solar radii, has revealed that switchbacks are a prominent feature of the nascent solar wind. My research work aims at understanding how waves, turbulence and particles evolve in the solar wind and what determines their observed properties. To this aim I have developed and helped to develop fluid and kinetic numerical models able to simulate plasma dynamics in an expanding medium. With this model, called the expanding box, it is possible to follow the evolution of the plasma, waves and turbulence with time and with distance from the sun, and use the numerical results as a point of comparison with in-situ observations.
​
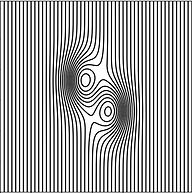

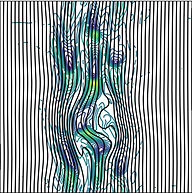
Predicted time evolution in a 2D time domain of a magnetic field kink (switchback) similar to those observed in the solar wind. Black lines represent magnetic field lines and colors represent plasma density fluctuations. The initial kink unfolds and decays by resonating with density fluctuations.
Magnetic field reconnection in quasi-ideal plasmas
​
Magnetic field reconnection is a process whereby magnetic energy is released locally to the plasma in the form of heat and kinetic energy via some mechanism of magnetic dissipation that allows for a change of magnetic field line connectivity. For this reason, magnetic reconnection is considered one of the most important mechanisms of energy conversion and magnetic field reorganization acting from space to the laboratory. It is believed to be at the heart of powerful and explosive events such as solar flares, coronal mass ejections, geomagnetic storms, and disruptive instabilities in tokamaks. In the non-collisional or weakly collisional plasmas typical of many astrophysical and laboratory environments, bulk plasma motions tend to form thin current sheets spontaneously in extremely localized regions. Quasi-singular current sheets may therefore set up naturally as a reservoir of free magnetic energy until they relax once magnetic reconnection is enabled — often explosively — inside these boundary layers. The research work I conduct addresses the dynamics of thin current sheets and boundary layers, what determines the onset of explosive reconnection, and the subsequent evolution of fields and particles in reconnecting current sheets. To this aim I adopt an analytical approach and numerical codes to model forming current sheets and to investigate their evolution in time, from the conditions that "turn on" reconnection to the complete disruption of the initial sheet.
​
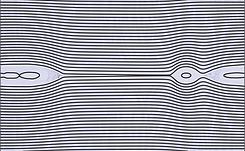

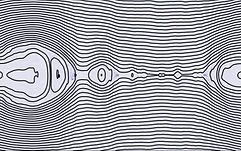
Time evolution of a reconnecting current sheet. The colors represent the current density intensity, and the black lines the magnetic field lines. The current sheet, localized at the center of the domain, is unstable to a reconnecting instability (tearing mode) that breaks it up into X and O points (magnetic islands.)
Kinetic instabilities and wave-particle interactions in non collisional plasmas​
​
​
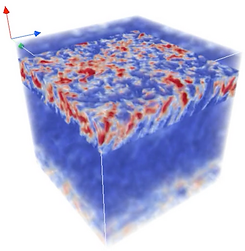
Simulation of a steepened wave front that interacts with protons generated from an initial broadband spectrum of Alfvénic fluctuations (simulation performed with CAMELIA)
Many natural and laboratory environments are characterized by non-collisional plasmas, that is, plasmas whose typical system size is smaller than the mean distance travelled by a particle before it can undergo a collision with another particle. As a consequence, and in contrast to "standard" gases, non-collisional plasmas are often found far from thermal equilibrium. For example, particle distributions observed in the solar wind or in our magnetosphere display many non-thermal features such as beams, supra-thermal populations, and pressure anisotropies that can provide the free energy for the onset of kinetic instabilities and enhance wave-particle interactions. These in turn can play a crucial role in the thermodynamics of the plasma by enhancing heating and transport, as well as by affecting the evolution of the turbulent cascade. By making use of extended fluid and kinetic models my research addresses how kinetic instabilities and wave-particle interactions impact the macroscopic properties of the plasma.